Abstract
The lung-on-a-chip is a clear, flexible microdevice that mimics the structure and function of the human lung. The size of a USB memory stick, the chip contains tiny hollow channels lined by living human lung cells. Inside the chip, realistically arranged cell layers are exposed to a flow of nutrients and air, as well as the mechanical forces of breathing. This microfluidic network represents the minimal functional unit that recapitulates the organ-level behavior of the lung. As a test bed for developing new pharmaceutical drugs, the lung-on-a-chip has demonstrated potential to replace current preclinical drug-testing methods, such as cell culture or animal models, which are often unreliable. The lung-on-a-chip is part of a larger family of organs-on-chips, which recreate the microenvironments experienced by cells of different organs in the human body. Future achievements in the organ-on-a-chip field will lead this promising effort to improve the effectiveness of the pharmaceutical drug development process.
Introduction
Welcome to the future of pharmaceuticals- where new drugs for treating lung conditions can be tested on synthetic human lung cells that live and breathe inside a microchip. In the past decade, researchers have pushed innovation by bridging tissue engineering, microfluidics, and materials science to create flexible polymer chips lined with living human cells that model the function of real human organ systems. These “organs-on-chips” are around the size of a USB memory stick [1], but don’t be fooled – each tiny chip houses a carefully-engineered microsystem with the potential to transform the paradigm of pharmaceutical research and development.
Wyss Institute Human Lung-on-a-Chip from Wyss Institute on Vimeo.
The Drug Problem
The process of bringing a new drug to market is not only expensive, but also high-risk. In 2016, the average cost of successfully developing a single drug in the United States was estimated to be over $2.5 billion, representing a 166% increase since 2013 [2]. An important factor in determining cost is the clinical success rate, demonstrating the probability that a new, clinical trial drug will eventually be approved. In 2013, the clinical success rate was 21.50%; in 2016, it dropped to 11.83% [2] – for every new drug that is approved, about nine others fail in the clinical stage.
Why do so many potential new drugs fail? A major roadblock arises from the limitations of traditional models used for pre-clinical testing. Before new drugs are tested on humans in clinical trials, they are analyzed extensively in 2-D cell cultures and animals. Although these trials reveal important information on the potential effects of these drugs in humans, the results are not necessarily directly translatable to human trials due to fundamental differences in biological systems between cell culture, animal, and human models [1,4]. For example, in cell cultures, flat layers of human cells grown on petri dishes often die or behave differently when compared to typical growth inside the human body [4].
Enter organs-on-chips: flexible, translucent microchips that mimic the architecture and function of human organs such as the lung, heart, or liver. With these new models, molecular-scale activity inside living cells, within the context of a human tissue or organ, can be watched in real time [1].
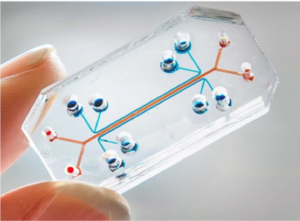
What’s in a Chip?
The word “chip” in organ-on-a-chip stems from its fabrication process, which is similar to the process used to create computer microchips [5]. Following a technique called “soft lithography”, the pattern for an organ-on-a-chip is etched into a silicon chip and then replicated in polydimethylsiloxane (PDMS), a biocompatible and flexible polymer. This is accomplished by pouring liquid PDMS onto an etched silicon template and curing the PDMS into a clear, rubber-like material [5]. The resulting PDMS replica contains the micro-scale channels and chambers that make up the organ-on-a-chip’s structure.
Organs-on-chips are designed to mimic human organs as accurately as possible, and each organ system poses its own unique design challenges. In the lung-on-a-chip, the challenge lies in replicating the blood-air barrier of human lungs, where gases are exchanged between tiny blood vessels, called capillaries, and air sacs, called alveoli. The lung-on-a-chip imitates this barrier with a porous, 10-μm-thick membrane made of PDMS [6]. The porous membrane separates an upper and lower cell channel, where alveolar cells and capillary cells grow on opposite sides, as in a real human lung (Fig. 2). Through a 3-D system of microchannels built into the PDMS chip, the alveolar cells are exposed to air on one side while they are fed nutrients from the “blood” channel on the other side [6]. This network of tiny channels is an example of the microfluidic technology that allows organs-on-chips to mimic the structure and critical functions of their real-life counterparts.
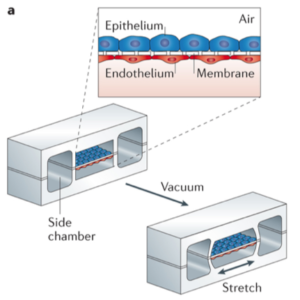
The human lung, of course, is not a static organ. Due to the motion of breathing, lung tissue is constantly being stretched in a cyclical pattern. The lung-on-a-chip captures the effects of this stretching by applying a similarly cyclical pattern of vacuum suction to a pair of hollow side chambers next to the main cell channels, as seen in Fig. 2. When the vacuum suction is applied, the resulting negative pressure mechanically stretches the cell layers in the main chamber, realistically simulating physical forces that occur in the lungs during breathing [6].
A Sea of Possibilities
The applications of the lung-on-a-chip are seemingly endless. Its 3-D network of channels allows researchers to push small quantities of chemicals through the chip and closely observe changes in cells under a microscope. As Dr. Donale E. Ingber, of the Wyss Institute at Harvard University, suggests, it is “an excellent test bed for pharmaceuticals” [1] and has been used to the study the absorption, distribution, metabolism, elimination and toxicity of chemicals [5].
Due to the lung-on-a-chip’s realistic structure and capacity to simulate the physical forces of breathing, made possible by the 3-D microfluidic channel system. For example, in an experiment that exposed the lung-on-a-chip to silica nanoparticles, it was found that the mechanical forces of breathing at the blood-air barrier increase the absorption of silica nanoparticles from the air channel to the blood channel [6].
Furthermore, the lung-on-a-chip can be used to model human diseases such as pulmonary edema: the accumulation of excess fluid in the lungs. Pulmonary edema can occur in patients with cancer who undergo chemotherapy that uses interleukin-2, a signaling molecule of the immune system [7]. Researchers administered interleukin-2 into the blood channel of the lung-on-a-chip, resulting in fluid leakage across the porous membrane into the air channel (Fig. 3) that mimics the disease in a real human lung [8]. This led to the discovery that a current drug candidate for a different condition completely inhibits interleukin-2–induced pulmonary edema [9]. By realistically modeling diseases, the lung-on-a-chip has become a powerful tool for discovering new therapeutics or novel applications of pre-existing ones. In the future, the lung-on-a-chip may play a similar role in uncovering new possibilities for preventing life-threatening conditions.
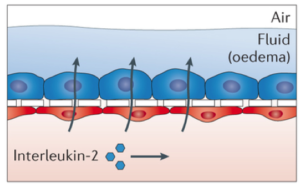
In the pharmaceutical industry, the lung-on-a-chip continues to show promise: Emulate, Inc. and Janssen Pharmaceuticals of Johnson & Johnson are using it to develop therapeutic drugs for pulmonary thrombosis [1], a condition that occurs when an artery in the lung becomes blocked by a blood clot. The organs-on-chips technology has been advancing Janssen’s clinical goals and “3Rs” program: reduction, refinement and replacement of animal testing [10].
Looking Ahead
As the field of organs-on-chips gains traction among researchers, the lung-on-a-chip will no doubt continue to find exciting new applications. One vision that Ingber has for these chips involves personalized medicine using stem-cell technology. Using the cells of people from specific genetic subpopulations, researchers could build personalized chips for drug testing. He describes: “Imagine you have a group of [people with asthma] who are female, belong to a particular ethnic group, and all have a higher sensitivity to smoke inhalation. Maybe you could develop a drug just for that small group, using the women’s personalized chips” [1].
The lung-on-a-chip not only stands alone as a model of the lung, but also supports a model that represents all of the organs in the human body as interconnected compartments [6].
Ingber’s team is interested in ultimately creating a “human-body-on-a-chip”, composed of 10 different human organs-on-chips linked together by flowing human blood or nutrient-containing liquid through a series of microchannels (Fig. 4). In this proposed network, reactions in one organ-on-a-chip could lead to an effect in another, replicating whole body-level responses. The team has already figured out how to couple some of these organs together; for example, they are able to watch a drug be absorbed by a gut-on-a-chip and then metabolized by a liver-on-a-chip [1]. In the future, scientists hope to add more organs, such as the lung, kidney, skin, eye, and blood-brain barrier, to the mix [10].
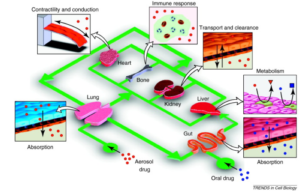
Of course, the process of making exciting new ideas for organs-on-chips into a reality does not come without technical challenges. For example, researchers are still looking for better ways to grow robust, consistent cell layers inside the microfluidic channels, prevent microbial contamination, and generate precise tissue structure-function relationships. Additionally, existing organs-on-chips would require a much longer time frame to investigate chronic diseases [5]. Furthermore, the PDMS material with which chips are made of poses a challenge. Although PDMS is biocompatible and easy to see through, it tends to absorb small organic compounds, which can limit the ability to test certain drugs. It may be difficult to find an alternative material that does not absorb small drug molecules, yet maintains the benefits of PDMS at a low cost [5]. Finally, some systems of the human body are harder to replicate than others. For example, organs-on-chips are not yet able to capture the more complex behaviors of the endocrine, skeletal, and nervous systems [5]. Researchers must find the balance between complexity and practicality: increasing the complexity of an organ-on-a-chip can improve its relevance, but will also present additional limitations to its practical operation [8].
Although organs-on-chips have demonstrated tremendous potential, there is a long road ahead with challenging obstacles to overcome. As innovative ideas continue to push the technology forward, we may one day see its widespread application in the pharmaceutical industry and beyond, bringing safer, more effective drugs to those in need.
References
[1] L. Levis, “Mimicking Organs”, Harvard Magazine, pp. 12-14, 2016. [Online]. Available: http://harvardmagazine.com/2015/12/mimicking-organs.
[2] J. DiMasi, H. Grabowski and R. Hansen, “Innovation in the pharmaceutical industry: New estimates of R&D costs”, Journal of Health Economics, vol. 47, pp. 20-33, 2016.
[3] M. Cimons, J. Getlin and T. Maugh II, “Cancer Drugs Face Long Road From Mice to Men”, Los Angeles Times, p. A1, 1998.
[4] E. Dougherty, “Living, breathing human lung-on-a-chip”, Harvard Gazette, 2010. [Online]. Available: http://news.harvard.edu/gazette/story/2010/06/living-breathing-human-lung-on-a-chip/.
[5] S. Bhatia and D. Ingber, “Microfluidic organs-on-chips”, Nat Biotechnol, vol. 32, no. 8, pp. 760-772, 2014.
[6] D. Huh, “A Human Breathing Lung-on-a-Chip”, Annals ATS, vol. 12, no. 1, pp. S42-S44, 2015.
[7] E. Conant, K. Fox and W. Miller, “Pulmonary edema as a complication of interleukin-2 therapy”, American Journal of Roentgenology, vol. 152, no. 4, pp. 749-752, 1989.
[8] E. Esch, A. Bahinski and D. Huh, “Organs-on-chips at the frontiers of drug discovery”, Nature Reviews Drug Discovery, vol. 14, no. 4, pp. 248-260, 2015.
[9] D. Huh, D. Leslie, B. Matthews, J. Fraser, S. Jurek, G. Hamilton, K. Thorneloe, M. McAlexander and D. Ingber, “A Human Disease Model of Drug Toxicity-Induced Pulmonary Edema in a Lung-on-a-Chip Microdevice”, Science Translational Medicine, vol. 4, no. 159, pp. 159ra147-159ra147, 2012.
[10] Emulate, Inc., “Emulate Announces Strategic Collaboration with Johnson & Johnson Innovation to Use Organs-on-Chips Platform to Better Predict Human Response in Drug Development Process”, 2015.